3dna Download
We present a comprehensive software package, 3DNA, for the analysis, reconstruction and visualization of three‐dimensional nucleic acid structures. Starting from a coordinate file in Protein Data Bank (PDB) format, 3DNA can handle antiparallel and parallel double helices, single‐stranded. To install 3DNA you will first have to download the software from: The webpage will ask you to register before allowing you to download the program. Once registered you'll be able to download the file which corresponds to your operating system.
3DNA is a versatile, integrated software system for the analysis, rebuilding, and visualization of three-dimensional nucleic-acid-containing structures. The software is applicable not only to DNA (as the name 3DNA may imply) but also to complicated RNA structures and DNA-protein complexes. In 3DNA, structural analysis and model rebuilding are two sides of the same coin: the description of the structure is rigorous and reversible, thus allowing for its exact reconstruction based on the derived parameters. 3DNA automatically detects all non-canonical base pairs, base triplets and higher-order associations (collectively termed multiplets), and coaxially stacked helices; provides a comprehensive collection of fiber models of regular DNA and RNA helices; generates highly effective schematic presentations that reveal key features of nucleic-acid structures; performs undisturbed, and have facilities for the analysis of molecular dynamics simulation trajectories. It is a representative of what would become the brand new version 3 of 3DNA. DSSR consolidates, refines, and significantly extends the functionality of 3DNA v2.x for RNA structural analysis.
An is a location in DNA or RNA where a purine or pyrimidine base is missing. It is also termed an AP site (i.e., apurinic/ apyrimidinic site) in biochemistry and molecular genetics. The abasic site can be formed either spontaneously (e.g., depurination) or due to DNA damage (occurring as intermediates in base excision repair). According to, “It has been estimated that under physiological conditions 10,000 apurinic sites and 500 apyrimidinic may be generated in a cell daily.” In DSSR and 3DNA v2.x, nucleotides are recognized using standard atom names and base planarity.
Thus, abasic sites are not taken as nucleotides (by default), simply because they do not have base atoms. DSSR introduced the -abasic option to account for abasic sites, a feature useful for detecting loops with backbone connectivity. For example, by default, DSSR identifies one internal loop (no. 1 in the list below) in PDB entry. With the -abasic option, two internal loops (including the one with the abasic site C.HPD18, no. 2) are detected. List of 2 internal loops 1 symmetric internal loop: nts=6; 1,1; linked by #-1,#1 summary: 2 1 1 B.1 C.24 B.3 C.22 1 4 nts=6 GTATAC B.DG1,B.DT2,B.DA3,C.DT22,C.DA23,C.DC24 nts=1 T B.DT2 nts=1 A C.DA23 2 symmetric internal loop: nts=6; 1,1; linked by #1,#2 summary: 2 1 1 B.6 C.19 B.8 C.17 4 5 nts=6 CTTA?G B.DC6,B.DT7,B.DT8,C.DA17,C.HPD18,C.DG19 nts=1 T B.DT7 nts=1?
C.HPD18 Note that C.HPD18 in is a non-standard residue, as shown in the HETATM records below. Since the identity of C.HPD18 cannot be deduced from the atomic records, its one-letter code is designated as? DSSR deliberately makes a distinction between ‘stem’ and ‘helix’, as shown below: a helix is defined by base-stacking interactions, regardless of bp type and backbone connectivity, and may contain more than one stem. A stem is defined as a helix consisting of only canonical WC/wobble pairs, with a continuous backbone.
By definition, a helix or stem consists of at least two base-pairs with stacking interactions. Helix is more inclusive and may contain more than one stem.
This differentiation between ‘helix’ and ‘stem’ naturally leads to the definition of coaxial stacking, another widely used yet vaguely specified concept. Again, the abstract notion can be best illustrated with a concrete example. In the classic yeast phenylalanine tRNA ( PDB id: ), DSSR identifies that two stems the acceptor stem (right) and the T stem (left) are coaxially stacked within one double helix. See the figure below. In the above schematics cartoon-block representation, each Watson-Crick base pair is rendered as a single, long rectangular block.
Base identities of the G–U wobble, and the two non-canonical pairs (left terminal) are illustrated separately, with a larger block size for purines (G and A), and a smaller size for pyrimidines (C, U, and T). I picked up ‘stem’ as a more specialized duplex because it is widely used in the RNA stem-loop structure, and in describing the four ‘paired regions’ of the classic tRNA cloverleaf secondary structure. On the other hand, ‘helix’ is (to me at least) a more general term, and thus more inclusive. It is worth noting that other terms such as ‘arm’, ‘paired region’, or ‘helix’ etc. Have also been used interchangeably in the literature to refer what DSSR designated as ‘stem’. As a side note, the basic algorithm for identifying helixes/stems in DSSR is also applicable for detecting G-quadruplexes.
The same idea of ‘helix’ or ‘stem’ also applies here (see figure below for PDB entry: ). Indeed, as of v1.7.0-2017oct19, DSSR contains a new section for the identification and characterization of G-quadruplexes. DSSR is “an integrated software tool for dissecting the spatial structure of RNA”. It excels in consolidating the diverse pieces together via a coherent framework, readily accessible in a solid software product.
DSSR may well serve as a cornerstone in RNA structural bioinformatics and would facilitate communications in the broad areas related to nucleic acids structures. G-quadruplexes (hereafter referred to as G4) are a common type of higher-order DNA and RNA structures formed from G-rich sequences.
The building block of G4 is a tetrad of guanines in a cyclic planar alignment, with four G+G pairs (cW+M type, see Figure below). A G4 structure is formed by stacking of G-tetrads and stabilized by cations at the center of the layers. G4 structures are polymorphic: the four strands can be parallel or anti-parallel, and loops connecting them can be of different types: lateral (edgewise), diagonal, or propeller (double-chain reversal). Moreover, G4 structures can be intra- or intermolecular, and even contain bulges.
From its initial releases, DSSR was able to detect G-tetrads, and listed them in a separate section. As of v1.7.0-2017oct19, DSSR has integrated existing features and created a new module to automatically identify and fully characterize G4 structures. The underlying algorithms have been further refined in v1.7.1-2017nov01, which was tested against all nucleic-acid-containing structures in the PDB. Characterizations of three representative G4 examples ( PDB entries 2m4p, 2hy9, and 5hix) are shown below, illustrating salient features (e.g., different types of loops) automatically extracted by DSSR. In addition to base pairs, DSSR also automatically detects higher-order base associations.
They are generally termed multiplets, consisting of three or more co-planar bases arranged together via H-bonding interactions. The simplest multiplets are base triplets. For example, the yeast phenylalanine tRNA ( PDB entry ) contains four base triplets, as shown below: The well-known (types I and II) are also multiplets of three bases.
Similarly, the G-tetrad where four guanine bases associate via Hoogsteen H-bonding to form a square planar structure is also a special multiplet. The G-tetrad is the building block of the. As of v1.7.0-2017oct19, DSSR can automatically identify and characterize G-quadruplexes (see the ). The DSSR algorithm for detecting multiplets is generally applicable. It can identify as many co-planar bases as available in a given structure. Shown below is an octad, consisting of a G-tetrad in the middle and four Us on the peripheries. The octad is derived from PDB entry using atomic coordinates from biological assembly 1 and 3.
Caption: 3D interactive visualization of selected RNA structural features enabled by the DSSR-Jmol integration. Clockwise from upper left: Structure of the xpt-pbuX guanine riboswitch in complex with hypoxanthine ( PDB id: 4fe5) in ‘base blocks’ representation. The three-way junction loop encompassing the metabolite (in space-filling representation) is color-coded by base identity: A, red; C, yellow; G, green; U, cyan. The loop-loop interaction (a kissing-loop motif) at the top is highlighted in red (upper left corner). Structure of the Thermus thermophilus 30S ribosomal subunit in complex with antibiotics ( PDB id: 1fjg) in step diagram. The 16S ribosomal RNA is color-coded in spectrum with the 5′-end in blue and the 3′-end in red (upper middle).

Structure of the classic L-shaped yeast phenylalanine tRNA ( PDB id: 1ehz) in step diagram, with the three hairpin loops highlighted in red and the 2,1,5,0 four-way junction loop in blue (upper right corner). Structure of the Pistol self-cleaving ribozyme ( PDB id: 5ktj), showcasing (in red) the horizontal helix in space-filling representation. The helix is composed of six short stems stabilized via coaxial stacking interactions (bottom). The DSSR-Jmol integration bridges the DSSR command-line analyzing tool and the Jmol molecular viewer seamlessly together via the standard JSON interface. Now users can select DSSR-derived RNA structural features (such as base pairs, double helices, various loops, etc.) and visualize them in novel representations in Jmol interactively.
Moreover, fine-grained characteristics of these features can be queried via the Jmol SQL for DSSR. The DSSR-Jmol integration fills a gap in RNA structural bioinformatics, and brings RNA visualization to an entirely new level.
The web interface is fully functional and easy to use, serving a huge user base of researchers, educators, and students alike. Featured as the cover image of the 2017 NAR web-server issue, DSSR's publicity would surely increase through the DSSR-Jmol integration. Additionally, I've written a new post (on the 3DNA Forum) that provides the scripts and datafiles used to create. I am pleased to announce the (advance online, May 3, 2017) publication of a new paper titled in Nucleic Acids Research ( NAR).
Co-authored by Robert Hanson (Jmol) and me ( DSSR), the article will appear in the July 2017 web-server issue of NAR. Here are the key links related to the paper:. The DSSR-Jmol integration project was initiated in October 2013 when I approached Bob at a meeting organized by RCSB PDB at Rutgers.
Thereafter, we met only once in July 2014 in Paris. Over the years, we have mostly communicated via email, occasionally facilitated by Skype. Our work bridges the DSSR command-line analyzing tool and the Jmol molecular viewer together via a simple JSON interface and a powerful query language. Users can now select DSSR-derived RNA structural features (such as base pairs, double helices, and various loops) as easily as they can select protein alpha-helices and beta-strands. Moreover, fine-grained characteristics of these features can be queried via Jmol SQL for DSSR (see examples below). Notably, the novel representation styles (step diagram and base blocks) and coloring schemes bring RNA visualization to an entirely new level (see ).
Recently, while analyzing a from the PDB, I came across three weird entries. They are documented below, primarily for my own record. — “Structure of the KH domain of T- STAR in complex with AAAUAA RNA”. There are two alternative conformations for the six-nt AAAUAA RNA component, labeled A and B, respectively. Normally, the A/B alternative coordinates for each atom are put directly next to each other, and assigned the same chain id, as in for the phosphate group of G2669 on chain A.
In, however, the two alternative conformations (A/B) are separated into two chains: chain H for A, and chain I for B. — “The structure of the transition state analogue ‘ DCSN’ bound to the large ribosomal subunit of Haloarcula marismortui”. The three-nt fragment on chain 4 is in the 3’—5’ direction.
— “The crystal structure of m(6)A RNA with the YTHDC1 YTH domain”. The mmCIF file has a model number of 0, instead of 1 (as in other cases I am aware of).
3DNA is a versatile, integrated software system for the analysis, rebuilding, and visualization of three-dimensional nucleic-acid-containing structures. The software is applicable not only to DNA (as the name 3DNA may imply) but also to complicated RNA structures and DNA-protein complexes. In 3DNA, structural analysis and model rebuilding are two sides of the same coin: the description of the structure is rigorous and reversible, thus allowing for its exact reconstruction based on the derived parameters. 3DNA automatically detects all non-canonical base pairs, base triplets and higher-order associations (collectively termed multiplets), and coaxially stacked helices; provides a comprehensive collection of fiber models of regular DNA and RNA helices; generates highly effective schematic presentations that reveal key features of nucleic-acid structures; performs undisturbed, and have facilities for the analysis of molecular dynamics simulation trajectories. It is a representative of what would become the brand new version 3 of 3DNA.
DSSR consolidates, refines, and significantly extends the functionality of 3DNA v2.x for RNA structural analysis. 3DNA contains 55 fiber models compiled from literature, plus a derived RNA model (as of v2.1). To the best of my knowledge, this is the most comprehensive collection of regular DNA/RNA models. Please see for detailed structural features of these models and references. The 55 models are based on the following works:. Chandrasekaran & Arnott (from #1 to #43) — the most well-known set of fiber models. Alexeev et al.
(#44-#45). van Dam & Levitt (#46-#47). Premilat & Albiser (#48-#55) The utility program fiber makes the generation of all these fiber models in a simple, consistent interface, and produces coordinate files in either PDB or PDBML format.
Of those models, some can be built with an arbitrary sequence of A, C, G and T (e.g., A-/B-/C- DNA from calf thymus), while others are of fixed sequences (e.g., Z- DNA with GC repeats). The sequence can be specified either from command-line or a plain text file, in either lower, UPPER, or MixED cases. Once 3DNA in properly installed, the command-line interface is the most versatile and convenient way to generate, e.g., a regular double-stranded DNA (mostly, B- DNA) of arbitrary sequence. As of release v2.3-2016sept06, the C source code of the 3DNA software package is available. The code can be found in the $X3DNA/src folder of the distributed tarballs for Linux, Mac OS X, and Windows.
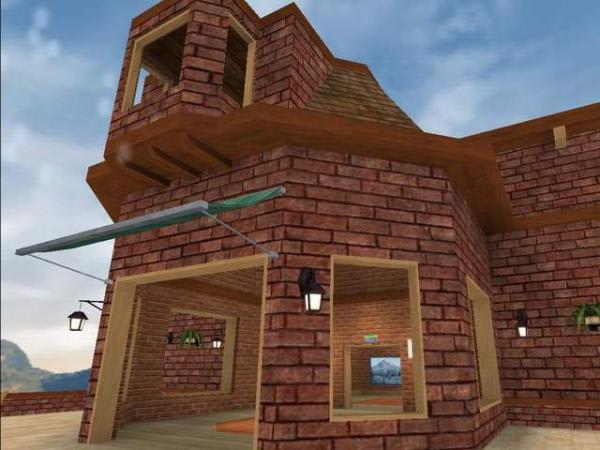
Since 3DNA is written in pure ANSI C, it can be compiled without changes on any platform with a modern C compiler. The original codebase of 3DNA was written around year 2000. Up until v2.3, the infrastructure of 3DNA has remained stable for 16 years. During the time, 3DNA has been widely adopted in other bioinformatics pipelines and cited over 1,500 times.
Volume Control
Over the years, I’ve received quite a few requests for 3DNA source code. However, due to complications of various factors (including software licensing), 3DNA had only been distributed in executable forms for the crucial C programs. Now, the C code of 3DNA is finally open source! As before, users need to register on the to download the software. The download page also includes x3dna-v2.0.tar.gz that accompanied the 2008, and x3dna-v1.5.tar.gz that corresponded to the. Other than minor revisions to pass strict gcc compiler options, the v1.5 and v2.0 codebases are kept as they were. 3DNA is backward-compatible as far as the key base-pair parameters are concerned.
Moreover, between v1.5 and v2.0, the command-line interface stays the same. The two previous versions are released for historical reasons. For example, one may notice some obvious “similarities” between 3DNA v1.5 and RNAView. The development of DSSR and SNAP will push 3DNA into a brand new version (v3), which contains significant changes in functionality and interface, and is no longer compatible with previous versions. I intend to keep 3DNA v2.3 in a ‘maintenance’ mode: no new features are planed, but bug reports and user questions will be promptly addressed on the 3DNA Forum, as always.
Making 3DNA open source should help further prompt its adoptions, and adaptations in structural bioinformatics of nucleic acids. There are numerous types of software licenses, but none of them seems to be a good fit for my purpose. As a result, I’ve come up with a permissive “ citation-ware” license with contents as below: 3DNA is a suite of software programs for the analysis, rebuilding and visualization of 3-Dimensional Nucleic Acid structures. Permission to use, copy, modify, and distribute this suite for any purpose, with or without fee, is hereby granted, and subject to the following conditions: At least one of the 3DNA papers must be cited, including the following two primary ones: 1. J., & Olson, W.
'3DNA: a software package for the analysis, rebuilding and visualization of three‐dimensional nucleic acid structures.' Nucleic Acids Research, 31(17), 5108-5121.
J., & Olson, W. '3DNA: a versatile, integrated software system for the analysis, rebuilding and visualization of three-dimensional nucleic-acid structures.' Nature Protocols, 3(7), 1213-1227. THE 3DNA SOFTWARE IS PROVIDED 'AS IS', WITHOUT EXPRESSED OR IMPLIED WARRANTY OF ANY KIND. Any 3DNA-related questions, comments, and suggestions are welcome and should be directed to the open 3DNA Forum (http://forum.x3dna.org/).
Upon user requests, I’ve recently introduced the -block-color option to DSSR, available as of v1.5.2-2016apr02. As its name implies, the -block-color option facilitate user customization of PyMOL rendered colors of the base rectangular blocks or their edges (e.g., the minor-groove) directly from the command-line. A simple example goes like this: -block-color='A blue; T red', which makes A colored blue and T colored red.
As detailed below, the new option is very flexible with regard to the specification of colors, bases, or some edges to highlight. Before that, a little background is in order.
Background info The DSSR cartoon-block representation follows the color convention of the original 3DNA blocview script, where A is red; C is yellow; G is green; T is blue; and U is cyan. If I remember correctly, the blocview coloring was based on the scheme adopted by the Nucleic Acid Database ( NDB). To allow for some flexibility, 3DNA includes a config file named $X3DNA/config/raster3d.par where users can change the values of the corresponding bases.
However, I do not know if any user has ever bothered to play around with the configuration file for customized base colors. Over the years, blocview-generated images have become popular, due to its simplicity, and (maybe more importantly) its endorsement by the NDB and PDB for nucleic acid structures. Via NDB, the blocview-generated images have also been used in and. Nevertheless, the blocview script has several dependencies: for protein or DNA/RNA backbone ribbons, render from for rendering, and for image processing. Moreover, the blocview script used by NDB/PDB is (likely to be) based on 3DNA v1.5, the last version before I left Rutgers in 2002. Over the years, 3DNA has been continuously refined, with significant changes introduced in v2.0 around 2008 to accompany the. Currently at v2.3, the codebase for 3DNA version 2 is in maintenance mode: the software will still be supported with identified bugs fixed, but no more new feature is planned.
3DNA version 3, as represented by DSSR and SNAP, is the way to go. DSSR has no third-party dependencies While creating DSSR, I set it as one of the design goals to make the program fully self-contained, without any third-party dependencies. Connections to other tools are clearly delineated via text files. If anything goes wrong, one can easily identify where the problem is.
Experience over the past few years has unambiguously proved the effectiveness of this zero-dependency approach. Other than being directly distributed with an operating system, DSSR is the easiest to get up and running. Moreover, DSSR can be easily integrated into other pipelines, including Jmol and PyMOL, among many other bioinformatics tools.
For the cartoon-block representation, DSSR produces.r3d files that can be loaded into PyMOL, mixed and matched with other visualization styles PyMOL has to offer. No more direct dependencies on MolScript, Raster3D, and ImageMagick as is the case for blocview. It is also worth mentioning that DSSR does not need PyMOL to run. DSSR and PyMOL are connected via.r3d files, a process which can be streamlined with the PyMOL plugin.
DSSR releases before v1.5.2-2016apr02 have the color coding of base blocks fixed within the source code, following the default style of blocview. Over the past few months, I’ve received at least two explicit requests on customizing the default colors of DSSR-generated base blocks. The -block-color option has been introduced for this purpose. Details of the -block-color option The general format of the option is as follows: -block-color='id color ; id2 color2.'
. id can be A, C, G, T, U, or the degenerated IUPAC code, including R, Y, N etc. See for details. id can also be minor, major, upper, bottom, wc-edge to specify one of the six faces of a 3D rectangular block. See for details. id can further be GC, AT, GU, pair, and variants thereof, to specify the colors of the corresponding long base-pair rectangular blocks.
color can be a common name (144 total), as specified in the website. For example, red, magenta, light gray etc. color can also be a single number in the range 0, 1 or 0, 255 to specify a shade of gray. DSSR repeat the number twice to get the RGB triple consisting of the same number.
color can further be a set of three space-delimited numbers to specify the RGB triple. Again, the number can be in 0, 1 or 0, 255. Moreover, the three numbers can be put in square brackets.
3dna Download Pc
For example -block-color='A 0 1 1' and -block-color='A 0 1 1' specify adenine to be colored with RGB triple 0 1 1 (aqua/cyan, corresponding to -block-color='A cyan'). More than one identity (bases) can be specified, separated by; (,:, or also works). Note: within the PyMOL dssrblock plugin, only or: can be used as a separator: comma (,) or semicolon (;) cannot be used as a separator within a PyMOL command argument (thanks to Thomas Holder for drawing this point to my attention).
Case does not matter when specifying id or color. So either ‘A’ or ‘a’, and ‘blue’ or ‘Blue’ or ‘ BLUE’ can be used to make adenine blue: -block-color='a blue'. Some example usages While the above description may appears to be quite complicated, the actual usage of the -block-color option is very straightforward. As always, the cases are best made with concrete examples, as shown below using the classic Dickerson B- DNA dodecamer.
# all bases in blue x3dna-dssr -i=355d.pdb -cartoon-block=orient -block-color='N blue' -o=355d-all-blue.pml # # all WC-pairs in red, with the minor-groove edge in 'dim gary' x3dna-dssr -i=355d.pdb -cartoon-block=orient -block-color='wc-pair red; minor dim gray' -o=355d-pair-minor.pml # # thymine (T) in purple, and the upper (+z) face in white # see Figure below, which shows the two bases in WC-pairs are anti-parallel x3dna-dssr -i=355d.pdb -cartoon-block=orient -block-color='T purple; upper 1' -o=355d-T-upper.pml. Recently I read the article titled by Krauss et al. I quickly ran DSSR on the corresponding PDB entry is. Not surprisingly, DSSR can automatically identify reported key structural features (see output file for details), including the TAT triplet at the quadruplex−duplex junction, and the three G-quartets.
Note that the result is based on biological assembly 1 in PDB file 5dww.pdb1 since the asymmetric unit contains four such molecules. List of 4 multiplets 1 nts=3 TAT 1:A.DT17,1:A.DA19,1:B.DT7 2 nts=4 GGGG 1:A.DG1,1:A.DG5,1:A.DG9,1:A.DG14 3 nts=4 GGGG 1:A.DG2,1:A.DG6,1:A.DG10,1:A.DG15 4 nts=4 GGGG 1:A.DG3,1:A.DG7,1:A.DG11,1:A.DG16 As its title suggests, however, this blog post is about the cartoon-block representations. Four styles of such schematics are shown below, which can all be easily generated using DSSR/PyMOL. In default style with base-pair blocks minor-groove highlighted top-face highlighted The cartoon-block representations possess unique features not seen elsewhere. With the help of the in PyMOL, they are extremely easy to generate. Such schematics are likely to become popular in illustrations of nucleic acid structures. Over the past couple of years, one of the most significant achievements of DSSR has been its integration into Jmol and PyMOL, two widely used molecular graphics programs.
None of the projects had been ‘ planned’, and I am honored to have the opportunities collaborating directly with Bob Hanson (Jmol) and Thomas Holder (PyMOL). The integrations make salient features of DSSR readily accessible to the Jmol and PyMOL user communities. Moreover, Jmol and PyMOL take different approaches to interoperate with DSSR, and so far they have employed separate features that the program has to offer. Key features of DSSR DSSR was implemented in strict ANSI C as a self-contained command-line program. The binaries for common operating systems (Mac OS X, Linux and Windows) are tiny (.